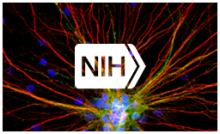
Unraveling neural contributors to position tracking errors in aging humans… A switch to shut off pain… Inducing torpor in mice in order to understand hibernation states… A novel and noninvasive improvement for optogenetics…
Utilizing virtual environments and machine learning to understand path integration in young and aged populations
Specific neurons within the brain work together to form a mental map of our environment, and path integration enables the tracking of an individual’s position in space via neural processes that link mental maps to self-motion cues. However, this sense of direction tends to diminish as we age. One research collaboration between the United States and Germany, led by Drs. Ila Fiete and Thomas Wolbers, seeks to understand how path integration deteriorates with age. The research team conducted an immersive virtual reality path integration experiment in human participants. In the study, young (~22 years old) and aged (~69 years old) participants were asked to track their own position and orientation as they were guided through a virtual environment. Next, the participants’ responses were analyzed by a new, powerful mathematical approach developed by the research team to identify sources of path integration errors. Dr. Wolbers told DZNE – German Center for Neurodegenerative Diseases, “With the help of our mathematical model, we were able to unravel the contributions of various sources of error and identify what distorts position tracking the most and what has little effect. Such sources of error have never been investigated at this level of detail.” Specifically, the model suggests that errors in path integration are primarily caused by the accumulation of “internal noise” in information processing, which is likely a consequence of inaccuracies in an individual’s perception of movement speed. Path integration errors were particularly prominent in aged individuals. This important work helps us to better understand age-related navigational impairments and may ultimately aid in the development of diagnostic tools that distinguish normal age-related orientation problems from those caused by disorders like dementia and Alzheimer’s disease.
Identification of pain-suppression neurons within the central amygdala
What if we could more effectively suppress chronic pain? Researchers at Duke University led by Dr. Fan Wang have uncovered one potentially potent therapeutic target in the fight against chronic pain – the central amygdala. Although the amygdala is classically known for its role in fear, anxiety, and learning, the researchers have recently demonstrated that a specific neuronal population composed of inhibitory GABAergic cells within the central nucleus of the amygdala plays a critical role in pain processes and effectively acts as a “pain off” switch. The team first identified these cells (termed CeAGA neurons) by using a neuronal marker of activity, c-Fos, following administration of general anesthesia in mice. Next, the researchers employed in vivo calcium imaging and a Fos-based viral-genetic method known as CANE (Capturing Activated Neuronal Ensembles) to better characterize CeAGA neuronal ensembles. Using optogenetic techniques, the researchers demonstrated that activation or silencing of CeAGA neurons bidirectionally altered pain reflexes (i.e., withdrawal responses to mechanical, thermal, and cold sensory tests) and pain-elicited self-caring behaviors (i.e., paw licking and face wiping) in awake mice. Indeed, when inhibitory CeAGA neurons were optogenetically activated, Dr. Wang told The New Daily, “It’s so drastic. [The mice] just instantaneously stop licking and rubbing.” The long-term effects of recurrent activation of this neuronal population are unknown, but these results could help in the identification and development of next generation painkillers for humans and have important clinical implications for patients suffering from chronic pain.
Identifying the brain region responsible for states of torpor
Torpor is a state of decreased activity that results from reductions in body temperature and metabolic processes and enables animals to survive periods of reduced food availability. While torpor is similar to hibernation in that it involves slowing of the body’s systems, it differs in that torpor is not a voluntary state, in contrast to hibernation. Torpor represents an adaptation that is a defining feature of mammalian and avian evolution, but its underlying neurobiology is poorly understood. A team of researchers from Harvard University led by Dr. Michael Greenberg recently shed light on this very issue using a model of fasting-induced torpor in mice. The researchers used excitatory Gq-DREADD (Gq-coupled Designer Receptor Exclusively Activated by Designer Drug) viral vectors in combination with a FosTRAP system (a tamoxifen-dependent form of Cre recombinase that is driven by Fos). Whole-brain imaging revealed which neurons and brain regions expressed the Gq-DREADD protein. Although simultaneous activation of several brain areas may be responsible for controlling torpor, the researchers revealed that the hypothalamus plays a major role in the regulation of torpor. Specifically, chemogenetic activation of the anterior and ventral portions of the medial and lateral preoptic area (avMLPA) of the hypothalamus resulted in rapid decreases in core body temperature and gross motor activity, which are behavioral characteristics of torpor. Further studies using single nucleus RNA sequencing suggested that avMLPA neurons that express Vglut2 or Adcyap1 regulate the decrease in body temperature that is associated with torpor. Altogether, these significant findings provide insight into how and why only certain species have the ability to enter torpor and the possibility of inducing this type of ancient adaptive behavior in other animal model systems.
Introduction of a new, highly sensitive optogenetic technique
Over the last decade, optogenetics has been a popular technique to manipulate neuronal activity with high temporal and spatial precision, but the invasiveness of optical fiber implantation is less than ideal. In an effort to improve this method, a multi-university team led by Dr. Guoping Feng at the Massachusetts Institute of Technology developed a step-function opsin with ultra-high light sensitivity (SOUL), which enables external light delivery and offers a minimally invasive tool for manipulating neuronal activity in both rodent and primate models. This method is particularly advantageous because, after activation, it allows the animals to be released from the light source while the neurons remain activated. Further, this method allows for synchronized spiking in all opsin-expressing cells at a firing rate that is determined by the researcher. First, the researchers developed and validated the SOUL method utilizing a combination of techniques, including Cre-inducible mouse lines, whole-cell recordings, and photocurrent stimulation. The researchers also demonstrate that SOUL has sufficient photosensitivity to allow for transcranial stimulation within a deep brain region, the lateral hypothalamus (LH). Transcranial stimulation increased the expression of an immediate early gene, c-Fos, demonstrating that it is sufficient to induce neural activity in the LH. The researchers then showed that food-deprived LH SOUL-expressing mice displayed reduced food consumption after SOUL activation by blue light, which were then inactivated by an orange light and food consumption was restored. They further showed that blue and orange light transcranial stimulation does not increase brain inflammation in the cortex. Dr. Feng’s team also demonstrated that this system works in macaques; here, the light was delivered above the dura using an optical fiber to activate deep cortical areas. SOUL was found to induce local field potential oscillations in the macaque cortex. Thus, SOUL may be a promising new tool in the field of neuroscience for the study of the relationship between local or circuit activity and behavior in a minimally invasive and temporally precise way.