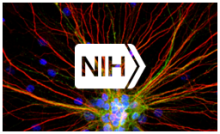
Improving two-color calcium imaging… Surprising evolutionary changes in interneuron types… Exploring how the basal ganglia multitasks behavior… Using mathematical models to study bat echolocation…
A new variant of the calcium indicator improves two-photon imaging
Two-photon (2P) calcium imaging has emerged as the leading method for in vivo brain imaging. However, the light sources commonly used (titanium-sapphire lasers and parametric oscillators) are quite costly, require frequent maintenance, and lack output power. One solution is to use inexpensive and powerful industrial lasers that have wavelengths around 1,000 nm. But to use these inexpensive lasers, new biosensors that excite above this wavelength are needed. To these ends, researchers have been working on creating new genetically encoded calcium indicators by taking advantage of mutations that can occur in green fluorescent protein (GFP) that convert it to a yellow fluorescent protein (YFP). Dr. Kaspar Podgorski and his team have created jYCaMP1 — a redshifted variant of jGCaMP7 — an optimized calcium indicator. Dr. Podgorski’s lab is a part of the Janelia Research Campus at the Howard Hughes Medical Institute. Their recent paper shows that jYCaMP1 outperforms jGCaMP7 in both mice and flies and enables improved two-color calcium imaging with red fluorescent protein-based indicators. When the researchers visualized activity within the mouse visual cortex, compared to jGCaMP7s, recordings from jYCaMP1s-labeled neurons were 1.8-fold as bright. Flies were also studied for a comparative analysis. When activity in the medulla of the optic lobe of flies was visualized, jYCaMP1s-labeled neurons showed 2.3-fold increases in brightness under 1,030 nm excitation compared to the jGCaMP7s-labeled neurons. One of the most exciting results of this study is that this new calcium indicator improves two-color imaging. This is because the shifted excitation spectrum of jYCaMP1 greatly improves the overlap with red genetically encoded fluorescent Ca2+ indicators, such as jRGECO1a, while still retaining well-separated emission with red sensors. This is critical because this new calcium indicator now allows for dual color 2P Ca2+ imaging with a single excitation laser. Technological advances like this are, without a doubt, the future of in vivo imaging of neural activity.
Evolution and the innovative variation of interneurons across species
While primates and rodents had a common evolutionary ancestor approximately 90 million years ago, profound differences exist in both behavior and cognitive capacity between primates and rodents. However, the cellular basis for these differences is unknown. Moreover, rodents such as mice are frequently used to understand cell types within the brain. Until now, it has been unclear whether or not these cell types have been conserved or if they have changed across evolution. In the present study, researchers sought to systemically characterize interneuron cell-type RNA expression across a variety of species. This study was led by senior researcher and professor at Harvard University, Dr. Steven McCarroll. Dr. McCarroll and his team used single-nucleus RNA sequencing to profile RNA expression in 188,776 individual interneurons across homologous brain regions of three primate species (i.e., human, macaque, and marmoset), mice, and ferrets. This paper shows that homologous interneuron types, readily identified by their RNA-expression patterns, varied in both abundance and RNA expression among ferrets, mice, and primates. As expected, this variance was lower among primates. Researchers predicted that the primate caudal ganglionic eminence (CGE) – a subcortical progenitor zone that gives rise to interneurons during neurodevelopment – would contain greater evolutionary novelties among interneurons because the CGE produces a larger proportion of these cells in primates than in rodents. However, they found that the divergence in the cortex, hippocampus, and striatum of the primate species all involved interneurons for which RNA-expression patterns indicated they originated from the medial ganglionic eminence (MGE) domain, which is evolutionarily older than the CGE domain. Further, the team found a primate interneuron cell-type that has no homologous counterpart in mice or ferrets in the striatum, and not the cortex. Overall, this research is important because it broadens our understanding of the evolution of interneurons. Further, these findings will facilitate research for the treatment of neuropsychiatric illnesses by helping scientists identify the species that best models cell types in the human brain.
Projections of the basal ganglia: beyond an integrative hub
There are primarily two models to explain the function of the basal ganglia, which are important nuclei involved in decision making and motor control. One model suggests this set of nuclei is a site of integration, whereas the second model suggests that projections from the basal ganglia are divided into topographical channels, each controlling a distinct behavior. The lab of Dr. Bernardo Sabatini investigated the latter hypothesis by using a unique experimental approach. One part of the basal ganglia, the striatum, has functionally discrete roles along the medial–lateral axis, with dorsal medial striatum and dorsal lateral striatum involved in goal-directed and habitual behaviors, respectively. Dr. Sabatini’s new paper suggests that this dichotomy might arise, at least partially, from distinct anatomical projections from the basal ganglia that allow parallel modulation of behavior. In other words, rather than integrating behavior on their own, the basal ganglia multitask through multiple feedforward projections to other regions. Researchers first used anterograde tracers to map the projection pathways of the basal ganglia. Next, using an optogenetic approach, they confirmed that specific regions within the striatum triggered a single behavior (licking or locomotion). Further, when the locomotion-inducing striatal region was stimulated while mice were licking, this did not interfere with licking. These findings support the canonical parallel model of basal ganglia activity, but also suggest that the integration and competition of information associated with specific behaviors occurs largely outside of the basal ganglia. Altogether, this work extends our understanding of the basal ganglia as a complex brain region with distinct neural projections that function independently. Understanding the functional composition of these circuits is a critical step toward a better understanding of basal ganglia-related disorders, such as Huntington’s disease and Parkinson’s disease.
Bats use echolocation to predict their prey’s movements
It is well established that bats use echolocation to track moving auditory objects, such as prey, within their environment. But are bats actually using predictive tracking strategies to anticipate the future? Dr. Cynthia Moss and her team at Johns Hopkins University study comparative neural systems and behavior using the big brown bat (Eptesicus fuscus) as a model organism. Dr. Moss and her “Batlab” team show in their new paper that bats integrate information across echo sequences to anticipate a target’s future position in a three-dimensional environment. To show this, the Batlab created a carefully controlled laboratory environment. They first trained bats to sit on a platform in this lab environment while the researchers manipulated the path of the insect the bats would chase. In this setting, the researchers could control the speed at which the insect moved, and high-speed cameras were used to track the movement of the bats. Then, they measured the direction of the bat’s head (i.e. sonar beam aim) and echolocation call rate as it tracked the moving target within its sonar field. The team then used four separate mathematical models to determine whether or not the bats used predictive tracking behaviors while chasing the insect target. Indeed, they found that bats use a predictive internal model to update tracking of a moving target. The results held true even when tested in a more naturalistic environment that included an object that the moving target could hide behind temporarily. By studying bats and other unique model organisms, we can better understand how humans and other animals use their own sensory information in order to make predictions about the future. Research such as this can have important implications about how individuals who are blind navigate within their environment and how we can create better devices to assist the visually impaired.