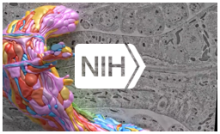
Improved method for optimizing resolution of magnetic particle imaging with important medical applications… New technique allows for successful 3D imaging of deep brain tissue in awake animals… Development of a stable deep brain stimulation electrode for long-term implantation…
Better image resolution in magnetic particle imaging (MPI) improves its competitive edge against MRI and CT scanning technologies.
Currently, when patients need an angiography performed to identify obstructions in blood vessels that could lead to heart attack or stroke, physicians use contrast computerized tomography (CT) or magnetic resonance imaging (MRI) scans. These require injection of a contrast agent such as iodine (for CT) or gadolinium (for MRI), both of which are processed through the kidneys. Unfortunately, this is not safe for people with chronic kidney disease. A potential alternative to CT or MRI is magnetic particle imaging (MPI), which detects superparamagnetic iron oxide (SPIO) nanoparticles, tracers that are safely processed by the liver. This imaging technique experiences no interference from tissue, provides images independent of source depth, and allows for images to be taken at much lower tracer concentrations. However, although MPI scans provide high contrast and sensitivity, the current spatial image resolution does not yet compete with that of CT or MRI.
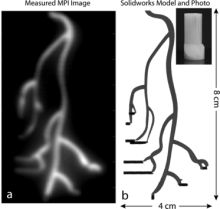
In a recent publication of Medical Physics, BRAIN Initiative grantee Dr. Steven Conolly (UC Berkeley) and colleagues experimentally investigated methods of improving MPI spatial resolution. Larger SPIO particle size can improve resolution, but their relaxation time (i.e. ability to flip magnetization and be detected) is slowed, which leads to blurred images. By adjusting a combination of scanning parameters, Conolly et al. were able to improve spatial resolution by up to 40%. These results would greatly improve the precision of MPI, providing a better imaging option that is also safe for people with chronic kidney disease. Further, if the resolution of MPI is able to surpass that of MRI, it could change the way human neuroimaging is done.
Researchers demonstrate improved in vivo deep tissue imaging technology in two types of tissue in mice: microglia in brain cortex and lymph nodes
Recording and analyzing biological dynamics, i.e. how cell systems change in space and time, requires high speed volumetric imaging at multiple time and physical scales. Limitations of current imaging techniques require a tradeoff between imaging speed and image resolution. For wide-field recording, technologies like light-field microscopy and temporal focusing multiphoton microscopy are most appropriate for their image speed and resolution, but are limited in their field of depth and only work in transparent biological systems such as cultured cells, C. elegans, and zebrafish larvae. Currently, laser scanning two-photon microscopy is the most popular method for in vivo deep tissue imaging, but it has slower imaging speed than wide-field recording methods, and still suffers from focus distortion in opaque tissues.
BRAIN Initiative grantee Dr. Meng Cui (Purdue University) and his lab previously developed two wavefront engineering techniques to address different aspects of improving tissue imaging. The first, Optical Phase-Locked Ultrasound Lens (OPLUL), is a high speed volumetric imaging system capable of imaging calcium activity in the neural network of the cerebral cortex of behaving mice. The second, Iterative Multiphoton Adaptive Compensation Technique (IMPACT), is capable of imaging through highly scattering tissue such as an intact mouse skull.
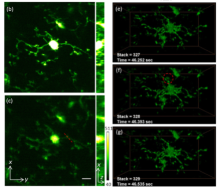
Dr. Cui and his colleagues’ recent publication in Optics Express combine these two techniques to create an improved technology for 3D imaging of cellular dynamics of deep tissue in awake animals, demonstrating its success in two applications in the mouse. After removing a window of the skull, the OPLUL and IMPACT system was capable of 3D-imaging the tissue at 400 µm under the surface of the brain with improved signal and low background fluorescence. Due to the improved spatial and temporal resolution, the group was able to record morphological changes in resting microglia, as well as capture rarely seen events – leukocytes circulating through the nearby blood vessels. Additionally, the OPLUL and IMPACT system recorded clear changes in distribution and morphology of mitochondria in a mouse lymph node even with respiration movement from the mouse. This new imaging technology will be an essential new tool in generating high spatial and temporal resolution for recording cell systems, particularly neural networks and cell-to-cell interactions, in the whole living brain.
Boron-doped diamond-based electrodes are superior to carbon fiber electrodes in longevity and strength while maintaining similar sensitivity
Deep brain stimulation (DBS) is currently used to treat several debilitating neurologic and neuropsychiatric disorders, such as essential tremor, Parkinson’s disease, depression, anxiety, and obsessive compulsive disorder, in patients who do not respond to other forms of therapy. One possible mechanism of action is via stimulating neurons to regulate or control neurochemical release, which may be abnormal in these disorders. DBS relies on pre-determined stimulation parameters in an open-loop configuration, but this requires external devices and can result in many adverse effects for the patient. A closed-loop system with neurophysiologic feedback to adjust the stimulation frequency and amplitude dynamically, i.e. a “smart” implanted device, would greatly improve effectiveness and reduce adverse effects. However, measuring stimulation-driven neurochemical release via fast-scan cyclic voltammetry (FSCV) is difficult because current electrodes are made of carbon fiber, which degrades quickly.
To solve this issue, BRAIN Initiative grantees Drs. Kendall Lee, Felicia Manciu, Jonathan Tomshine, and their Mayo Clinic colleagues developed a durable, synthetic, boron-doped diamond-based electrode and, in a recent publication in Frontiers in Human Neuroscience, characterized over 500 individual electrodes for their efficacy in human applications. Electrode sensitivity to dopamine was comparable to carbon fiber electrodes, but longevity and mechanical stability were far superior in the diamond-based electrode. Over a period of time equivalent to approximately 1 scan every 2 minutes for 20 years, the diamond-based electrodes only accrued a 16.1% loss of sensitivity while carbon fiber electrodes suffered an 89.4% loss in sensitivity, rendering the carbon fiber electrodes useless. Further, a scanning electron microscope showed no discernable changes in the diamond-based electrode, but the carbon fiber electrode was almost completely eroded. Under a force test, the carbon fiber electrodes snapped upon failure, whereas the diamond-based electrode tips merely bent, making them sturdier during implantation or removal. Proof of concept was conducted in vivo in rats, pigs, and humans already approved for DBS surgery: all three showed successful application of FSCV via diamond-based electrodes and detection of evoked changes in extracellular neurochemicals in the brain. This technological advance in electrode strength and longevity makes an FSCV-based, closed loop DBS system for chronic therapeutic implantation a practical possibility in the near future.
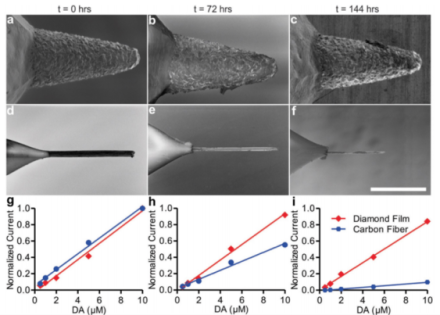