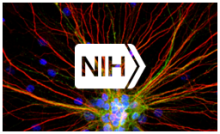
Remote, transgene-free magnetothermal regulation of adrenal hormones
Scientists can use implantable devices to modulate electrical activity within the body and current less invasive techniques lack precision and rely on transgenes – but what if there was an alternative, transgene-free noninvasive strategy? The robust, endogenous expression of a heat-sensitive cation channel, transient receptor potential vanilloid family member 1 (TRPV1), allows for the possibility of applying magnetothermal control to remotely modulate organ function without relying on transgenes. Dr. Polina Anikeeva and her colleagues at the Massachusetts Institute of Technology (MIT) developed a magnetothermal switch to wirelessly control the release of the adrenal hormones epinephrine and corticosterone in a genetically unaltered rat. After verifying that the TRPV1 receptor was highly expressed in the adrenal gland using immunofluorescent labeling procedures, researchers characterized the magnetic nanoparticles to determine if these particles were necessary and sufficient to trigger a robust calcium ion influx into TRPV1-expressing adrenal cells. First, they verified that the new magnetothermal control system worked in vitro and then in vivo. Altogether, this study demonstrates that these new magnetic nanoparticles can persist within tissue for up to six months and allow for chronic stimulation of the adrenal gland over days and weeks without damaging tissues or altering cellular function. Dr. Anikeeva and her team’s work paves the way for technological advancements in remote, noninvasive control of organ function and may help inform bioelectronic medicines in humans. Eventually, given the central role of adrenal glands in regulating the stress response in mammals, technology like this could one day be used to treat stress-related disorders by modulating peripheral organ function.
***
Performance in brain-computer interface learning is predicted by the organization of cortical areas
Brain-computer interface (BCI) learning can be used to improve neurofeedback devices that directly regulate an external device, but how can we improve on their usability? One way to improve BCI technology is to gain a better understanding of the neural processes that enable BCI control, such as neuroplasticity. Recently, Dr. Danielle Bassett at the University of Pennsylvania and her colleagues at Sorbonne Université in Paris, France, characterized the evolution of large-scale cortical network changes that occur during BCI training. Specifically, researchers predicted that BCI learning would be accompanied by a decrease in functional integration in cortical regions strongly associated with cognitive processing as BCI users transition from using a deliberate mental strategy to processing nearly automatically. To determine precisely how cortical connectivity changes, the researchers simultaneously recorded high-density electroencephalographic (EEG) and magnetoencephalographic (MEG) signals during BCI training, which consisted of four sessions over a period of two weeks. They found that at the end of the two weeks, event-related desynchronizations – which reflect sensorimotor brain activity – were more localized in the contralateral paracentral lobule, precentral gyrus, and superior parietal lobule. These regions are typically involved in hand motor tasks and motor imagery. Dr. Bassett and her team also observed that functional connectivity was reduced in the orbital part of the inferior frontal gyrus, an area involved in mental rotation and working memory. This area was also strongly predictive of BCI scores. Altogether, understanding the large-scale neural mechanisms underlying motor plasticity is fundamental to our understanding of the cognitive aspects of human learning and may inform the development of better machine learning interfaces in the future.
***
New shifts in dynamic and noninvasive human brain imaging technology
Human brain imaging has come a long way in the nearly 100 years since the discovery of the electroencephalogram (EEG), but every next step towards higher resolution processing is a step towards improving patient outcomes. Dr. Gregory Worrell from the Mayo Clinic in Minnesota and his colleagues from the Carnegie Mellon University in Pennsylvania created a novel non-invasive, high-spatiotemporal resolution source imaging approach to map the brain networks of individuals with epilepsy. Specifically, they demonstrated the effectiveness of the fast spatiotemporal iteratively reweighted edge sparsity (FAST-IRES) technique, which uses unbiased machine learning to estimate signal sources and activity across time. Here, they used this approach to estimate the epileptogenic zone (EZ), or the minimum amount of brain tissue that needs to be removed to stop seizures. The FAST-IRES technique was further validated through a direct comparison to intracranial EEG findings and surgical outcomes in 36 patients with focal epilepsy. In total, 1,027 spikes and 86 seizures were analyzed. Altogether, the new FAST-IRES approach could be applied to both medical and research settings to objectively and noninvasively, with high precision, acquire human brain imaging data via scalp high-density EEG recordings. FAST-IRES can work in collaboration with existing hardware to improve, for instance, the management of epilepsy. For more information, check out Carnegie Mellon University’s news article on this exciting technology.
***
A new regenerative peripheral nerve interface allows for real time control of prosthetic limbs in amputees
Restoration of movement is something that many upper limb amputees struggle with – but new advances in regenerative nerve interface technology allows for the real time control of prosthetic limbs. Here, Dr. Cynthia Chestek at the University of Michigan and her team reveal a newly designed, biologically stable regenerative peripheral nerve interface (RPNI). This RPNI is a peripheral nerve bioamplifier that produces high-amplitude surface electromyography (EMG) signals and can be used to control a prosthetic device. Its use also indicated that RPNIs can reduce phantom limb pain. This nerve regeneration interface was applied successfully even in cases where years had elapsed since the initial injury. Overall, the authors demonstrate in multiple independent cases of upper limb loss that these RPNIs might be an effective peripheral nerve interface for control of prosthetic devices. Dr. Chestek and her colleagues also demonstrate that RPNIs are a viable alternative to existing prosthetic control approaches that interface with intact muscles, where directly extracting efferent motor action potentials from the peripheral nerve is possible. A device such as this has the potential to revolutionize the future of clinical prosthetic technology, as well as greatly improve the quality of life for patients with limb loss. For more information, check out The Michigan Engineer News Center article on this innovative work.