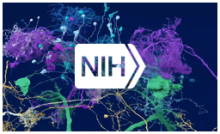
Fabrication and testing of implanted magnetic microcoils to stimulate neurons… Optogenetic-induced seizure model to enhance understanding of interneuron roles… New optical technique to identify voltage activity patterns in specific cell types…
Novel implantable magnetic microcoils stimulate cortical cells and consistently drive behavioral responses
Modulating neuronal activity through implanted devices, like electrodes and electrode arrays, offers potential relief from neurological disorders and opportunities for prosthetics that rely on brain-machine interfaces. Unfortunately, most implanted devices suffer from limitations including imprecise cellular activation (whether of cell types or passing fibers) and inflammation at the implant site. At Massachusetts General Hospital, Dr. Shelley Fried’s group proposes the use of novel magnetic microcoils – no larger than the width of a mouse cortical column – that can be safely and chronically implanted in the cortex to allow more targeted stimulation of cells to reliably elicit behaviors. To follow up on computational models of the coils, the group tested two coil designs in vitro, determining that the microcoils were most effective when oriented parallel to mouse pyramidal neurons and could stimulate regions as narrow as 60 µm at significantly reduced activation thresholds without spreading to passing axons. Dr. Fried’s team then implanted the coils in mouse motor and sensory cortices associated with whisking behaviors to illustrate the safety and efficacy of the designs when modulating whisker movement. These microcoils could offer a longer-lasting method of more selective cortical stimulation that can withstand the inflammation response relative to existing electrode-based technologies.
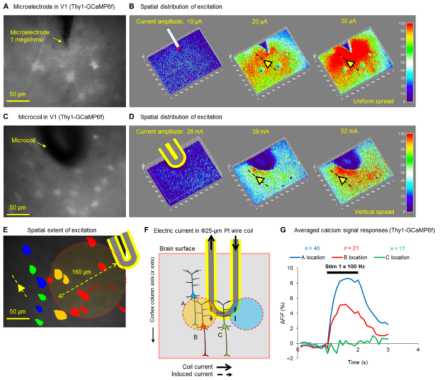
Optogenetics enables identification of distinct roles for interneuron subtypes in mouse model of seizures
Extensive work on the mechanisms underlying seizures and epilepsy has identified a role for GABAergic interneurons, but models of spontaneous seizure activity lack the temporal control necessary to parse cell-type specific roles in the genesis and maintenance of seizures. At the University of California, San Francisco, Dr. Vikaas Sohal’s lab employed optogenetic and photometric imaging techniques to reliably and repeatedly induce seizure activity in mice with high temporal precision, simultaneously record electrical activity using electroencephalogram (EEG) and cell-type specific calcium signals, and manipulate the activity of selected subtypes of GABAergic interneurons to modulate seizure activity. The group expressed channelrhodopsin in mouse primary motor cortex to induce seizure activity using a sequence of optogenetic stimuli with temporal specificity. Simultaneous with optogenetic seizure induction, they used fiber photometry to record from labeled interneuron subtypes expressing parvalbumin (PV+), somatostatin (SOM+), or vasoactive intestinal peptide (VIP+), as well as excitatory neurons in neocortex. They demonstrated that all of the interneurons activate within one second following seizure onset, excitatory neurons exhibit an approximately 10 s delay, and calcium signaling varies across cell types during seizure activity. To explore causal roles for the different interneurons before and during seizures, the group again relied on optogenetics to systematically inhibit each subtype. Inhibition of VIP+ interneurons contralateral to the seizure induction site increased seizure threshold, reduced seizure probability, and reduced seizure duration, while inhibition of PV+ and/or SOM+ interneurons inconsistently modulated seizure activity. Overall, this novel, time-selective model of optogenetically-induced seizures revealed the anti-seizure effect of inhibiting VIP+ interneurons, providing a novel potential therapeutic target for anti-epileptic therapies.
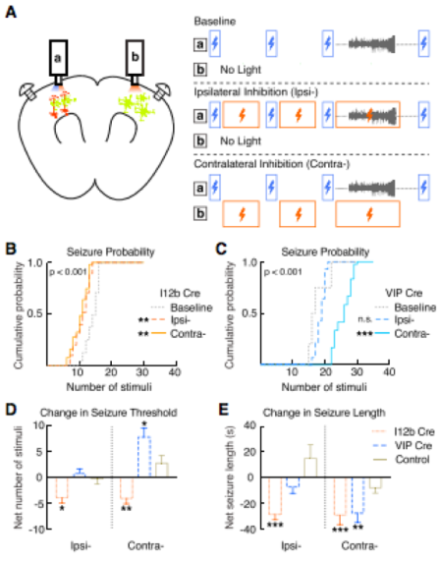
Transmembrane Electrical Measurements Performed Optically (TEMPO) records voltage activity of distinct cell types in freely moving animals with high sensitivity
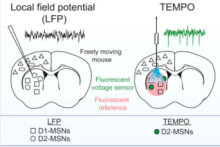
Techniques to measure the dynamics of neural activity, including intracellular recordings and genetically-encoded calcium level indicators, have advanced significantly, but researchers struggle to distinguish both spiking and oscillatory contributions of multiple cell types in a given brain region with high temporal sensitivity – particularly in behaving animals. Dr. Mark Schnitzer’s team at Stanford University describe an optical imaging technique, TEMPO, that is 10 times more sensitive than calcium indicators, records voltage signals near the physical sensitivity limits of optical imaging, and can distinguish hyperpolarization states of interspersed cell types with high fidelity. First, Dr. Schnitzer and colleagues validated TEMPO by measuring cortical and hippocampal oscillatory activity in anesthetized and awake, behaving mice, reproducing data well-established in studies using electroencephalogram (EEG) and local field potential (LFP) recordings. Next, the researchers selectively monitored voltage dynamics of two medium spiny neuron subtypes using transgenic animals, revealing two forms of synchronized hyperpolarization they could connect to distinct brain states in freely behaving mice, all previously unresolved by EEG or LFP. Taken together, these data identify TEMPO as a highly sensitive method to record neural voltage dynamics in specific cell types in awake and behaving animals at significantly higher resolution than current recording techniques.
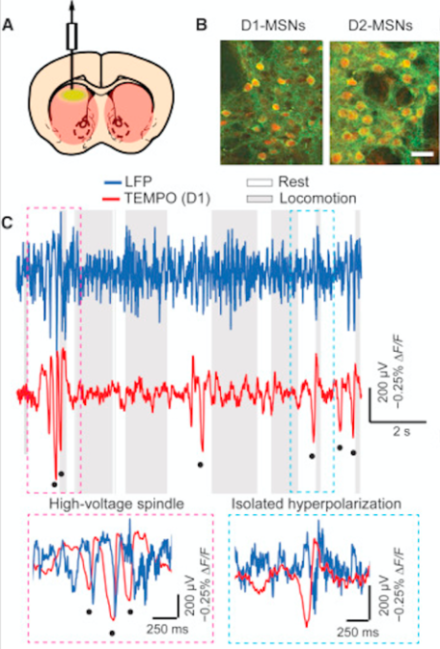