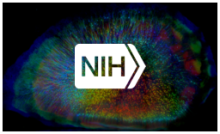
Widespread delivery of genes across the blood brain barrier…light-activated glutamate receptors become more specialized, used in vivo for first time…manipulations of intraocular pressure are used to measure intracranial pressure…researchers discover new types of retinal ganglion cells.
Designing a more efficient way to transfer genes to neurons throughout the brain
Small, harmless viruses called adeno-associated viruses (AAV) have been used over the past two decades to transport specific genes into the nuclei of cells. This delivery system can be used clinically to carry functional copies of genes into cell nuclei in individuals with genetic diseases. Clinical applications have been hindered by the inability of current AAVs to cross the blood brain barrier (BBB) and achieve widespread transfer across the brain. Caltech researchers, led by BRAIN Initiative grantee Dr. Viviana Gradinaru, created millions of genetic variants of AAV9, the most effective AAV for gene transfer. They then created an assay, described in a recent issue of Nature Biotechnology, to test the efficiency of these variants and found one, AAV-PHP.B, which crosses the BBB and transfers genes with 40 times the efficiency of AAV9.
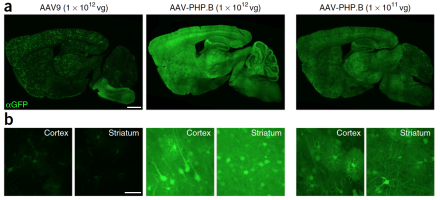
Researchers develop toolkit of light-activated glutamate receptors and demonstrate their use in vivo
Optogenetics—the ability to use light to manipulate the activity of neurons with genetically expressed light sensitive ion channels—is a powerful technique for mapping neural circuits and determining the roles populations of neurons play in a variety of behaviors. To understand the subcellular, molecular basis of brain function, however, requires the manipulation of natively expressed signaling proteins. A few years ago, BRAIN Initiative grantee Dr. Ehud Isaacoff and his UC Berkeley colleagues developed a series of light-activated glutamate receptors (LiGluR). The researchers describe in a recent Frontiers in Molecular Neuroscience paper how they have further engineered their LiGluRs based on known properties of glutamate receptor function to make designer variants for specific functions. In addition, the new paper reports the first in vivo use of the LiGluRs, which they demonstrated in mice.
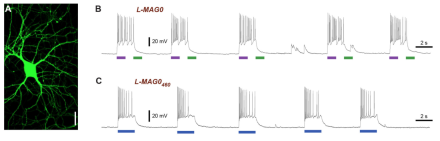
Modulating intraocular pressure helps researchers non-invasively measure intracranial pressure
Changes in intracranial pressure (ICP) are associated with several serious medical conditions, including traumatic brain injury, stroke, brain tumors, and glaucoma. Current techniques for measuring ICO are highly invasive, requiring either a spinal tap or a needle inserted into the brain. BRAIN Initiative grantee Dr. Craig Forest of the Georgia Institute of Technology and colleagues have designed, fabricated, and tested a system for measuring ICP non-invasively. The researchers’ system, described in a recent issue of PLOS One, takes measure pulsation of a blood vessel called the central retinal vein. This vein visibly pulsates when ICP and intraocular pressure (IOP) are normal. When ICP is high or IOP is low, it does not pulsate. The researchers manipulated IOP with injections of small volumes of liquid into the eye until the retinal vein stopped pulsating. The volume needed to achieve this was used to indicate ICP. More work is needed to make the measurement accurate enough for the clinic.
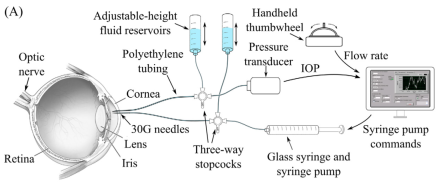
2-photon calcium imaging reveals functionally diverse retinal ganglion cells in mouse retina
In the visual system, photoreceptors transmit visual information to bipolar cells, which provide input to a variety of retinal ganglion cells (RGCs). Each type of RGC extracts specific features of the visual world for transmission to the brain. Previous experiments examining the anatomy of RGCs and their electrical responses to different kinds of visual stimuli have suggested that there are 15-20 unique types of RGC, each with a unique function. BRAIN Initiative grantee Dr. Thomas Euler, of the University of Tübingen in Germany, and his colleagues, used 2-photon calcium imaging to measure responses of more than 11,000 RGCs from 50 mouse retinas to a variety of visual stimuli. The researchers fed the resulting imaging data, which was recently published in Nature, into an unsupervised machine-learning algorithm that was designed to classify all of the cells into unique types. They discovered a minimum of 32, but possibly up to 49, separate RGC types.
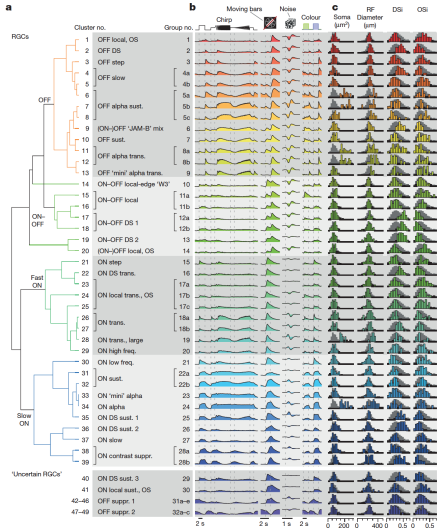